4 The Use of Concrete in Architecture: A Deep Dive into Foundation Design
Introduction
In this chapter, we explore the pivotal role of concrete in architecture, focusing on its use in foundation design. We delve into the properties of concrete that make it the material of choice for foundations, the principles of foundation design, and the application of concrete in different types of foundations. Modern innovations, maintenance strategies, environmental considerations, and case studies will also be examined to comprehensively understand the subject matter.
The history of concrete use in architecture traces back to ancient times, with the Romans being the first to employ it extensively. They utilized a primitive form of concrete to construct monumental architectural marvels, some of which still stand today. However, the understanding and use of concrete were lost during the Middle Ages, to be rediscovered in the 18th century. The 20th century saw a significant rise in its use due to the development of reinforced concrete, which offered higher strength and durability. Concrete’s versatility, cost-effectiveness, and structural properties make it a cornerstone material in modern construction, especially in foundation design.
Concrete plays a crucial role in foundation design due to its numerous attributes. Its compressive strength makes it ideal for bearing heavy loads, a fundamental requirement for foundations. The material’s durability ensures the longevity of the structure, given the foundation’s constant exposure to soil and moisture. Moreover, concrete is moldable, enabling the creation of foundations in various shapes to cater to diverse architectural requirements. Additionally, reinforced concrete, which combines concrete’s compressive strength with steel’s tensile strength, has broadened the scope of foundation design. Overall, concrete’s use in foundations is pivotal for the stability and durability of architectural structures.
Properties of Concrete
Concrete is a composite material composed primarily of water, cement, and aggregates, which are usually sand and crushed stone. From a chemical perspective, the process of hardening or ‘curing’ begins when water reacts with the cement—a process known as hydration. The resulting hardened mass provides the durability and strength concrete is known for. Physically, the properties of concrete can vary widely and depend on the ratio of its components and the size and type of aggregates used. Concrete’s most significant physical properties include high compressive strength, low tensile strength, density, and durability. The compressive strength of concrete, usually ranging from 20 to 40 MPa in residential construction, makes it an excellent material for load-bearing structures like foundations.
Types of Concrete and Their Specific Uses in Architecture
Various types of concrete cater to different architectural needs. Standard ready-mix concrete is commonly used for foundations, sidewalks, and driveways due to its strength and durability. High-performance concrete, with its enhanced characteristics such as high strength and durability, finds use in long-span bridges and high-rise buildings. Lightweight concrete, because of its reduced weight, is often used in the construction of partition walls and fire-resistant structures. Architectural concrete is preferred for aesthetic purposes because it provides a high-quality finish. Lastly, pervious concrete is employed in the construction of surfaces subject to water run-off, as it permits water to percolate through, reducing the risk of flooding.
Advantages and Limitations of Concrete
Concrete offers many advantages that have made it a fundamental material in construction. It provides high compressive strength, durability, and resistance to fire and water, making it ideal for use in foundations. Its ability to be cast into various shapes makes it versatile for design needs. Moreover, the raw materials for concrete are abundant and relatively inexpensive, making it cost-effective.
However, concrete also has some limitations. It has low tensile strength and is prone to cracking under tension unless reinforced. Concrete is also a significant contributor to CO2 emissions due to the energy-intense production of cement. Another disadvantage is the potential for shrinkage and creeps over time, which can lead to structural issues if not properly accounted for in the design. Despite these limitations, the benefits of using concrete in architectural applications, particularly in foundation design, are significant.
Foundation Design Basics
Role of the Foundation in a Structure
The foundation serves as the critical interface between a structure and the ground upon which it is built. The essential component transfers loads from the building to the ground, ensuring stability and structural integrity.
The foundation primarily fulfills two critical roles: load distribution and stability. It spreads the load of the structure over a broad area, reducing the intensity of the weight over any specific point and thus preventing over-stressing of the soil, which could lead to uneven settlement. This process involves the transfer of the structure’s dead load (the weight of the building components themselves) and the live load (additional forces such as those exerted by occupants, furnishings, and environmental factors like wind or snow).
In terms of stability, a well-designed foundation prevents movement of the structure, providing resistance against the uneven settlement, uplift, or lateral forces that might otherwise cause tipping or sliding. It is especially crucial in areas prone to earthquakes, high winds, or expansive soils. Therefore, designing a reliable foundation is of paramount importance in the overall stability and longevity of a structure.
Key Factors in Foundation Design
Designing a foundation requires careful consideration of several factors to ensure the stability, safety, and longevity of the structure. The primary factors are:
1. Type of Soil: The type and properties of soil significantly influence the choice of foundation. For instance, clayey soils with high plasticity can lead to significant volume changes with moisture variation and may require deep foundations, while sandy or gravelly soils with good bearing capacity are suitable for shallow foundations.
2. Load of the Structure: The load that the foundation needs to bear, including the dead load, live load, and environmental loads such as wind or snow load, must be accurately estimated. The foundation should be designed to distribute these loads evenly to prevent structural damage or failure.
3. Topography: The terrain where the structure is to be built impacts the foundation design. A sloping site or one with uneven ground may necessitate a specific foundation design, such as stepped or pile foundations.
4. Water Table Level: A high water table can affect the strength and stability of the foundation and lead to issues like dampness and instability. In such cases, waterproofing measures or a specific foundation type may be required.
5. Seismic Considerations: In earthquake-prone areas, the foundation design should accommodate lateral forces and prevent differential settlement or structural failure during seismic events.
6. Environmental Impact: Sustainable practices encourage minimal disturbance of the site and use of materials and techniques that reduce the carbon footprint and enhance energy efficiency.
7. Cost: Cost-effectiveness is crucial. The choice of foundation should balance the need for structural integrity and stability with economic feasibility.
Types of Foundations
The type of foundation selected for a structure depends primarily on the soil type, load of the structure, and site conditions. Here are the most commonly used types:
Shallow Foundations: These are placed near the ground surface, usually no more than the width of the footing below the surface. They are typically used when dealing with hard soil that has a high bearing capacity. Examples include:
- Strip Footing: This type of footing supports a line of loads, such as a wall, and is commonly used in load-bearing wall structures.
- Spread or Isolated Footing: This type supports a single column. It is generally square or rectangular and distributes the load over a larger area.
- Mat or Raft Foundation: This is used when the columns are close, and the loads are heavy, causing the strip or isolated footings to overlap. A mat foundation covers the entire area under a structure, distributing the load across a large surface.
Deep Foundations: These are used when the topsoil is weak or when the load of the structure must be transferred to a deeper, stronger layer of soil or rock. Examples include:
- Pile Foundations: They are long, slender columns made from steel, concrete, or timber, driven deep into the ground to transfer loads to stronger soil strata.
- Pier Foundations: Similar to piles, piers are designed to carry heavy vertical loads but are not driven into the ground. Instead, they are cast in place in a deep excavation.
Understanding these different types of foundations and their appropriate use cases is critical to successful foundation design.
The Use of Concrete in Different Types of Foundations
Shallow Foundations
Strip Footing
Strip footings, which support an entire line of loads such as load-bearing walls, often utilize concrete for its high durability and load-bearing capacity. Reinforced concrete, with its improved tensile strength, resists bending stresses and effectively carries the load from walls to the ground. Concrete’s moldability ensures the footing matches the wall’s specific dimensions, providing an optimized load distribution system.
Spread or Isolated Footing
Concrete’s adaptability is particularly useful in isolated footings, designed to support a single column. The footing, made wider than the column, ensures the load is spread over a larger area, preventing soil over-stressing. The malleability of concrete allows it to be poured and set to precise dimensions, while reinforcement improves the resistance to tensile and shear stresses.
Mat or Raft Foundation
Mat or raft foundations are large concrete slabs extending over a building’s entire area, supporting all columns and walls. Predominantly used when soil conditions are weak, concrete is crucial for its high compressive strength and castability into large, uniform slabs. The slab often includes two-way reinforcement to enhance its strength and durability.
Deep Foundations
Pile Foundations
Pile foundations often employ concrete piles due to their high compressive strength and durability. These piles, which can either be precast or cast-in-situ, withstand heavy loads and aggressive soil conditions. Concrete piles are typically reinforced to improve their tensile strength and effectively transfer the building load to a stronger, deeper soil stratum.
Pier Foundations
In pier foundations, deep holes are drilled and then filled with concrete and reinforcement, forming a robust and durable foundation. The process allows the load to be transferred to a greater depth, ideal for handling heavy loads or dealing with weak surface soils. Concrete’s high compressive strength and durability are key to the performance of these foundations.
Caissons
Caissons are similar to pile foundations but are larger in diameter and often filled with concrete after being placed into the excavation. Concrete caissons provide high load-bearing capacity and resist lateral loads, making them suitable for structures like bridges or piers. Reinforced concrete caissons further increase tensile strength, making them more robust.
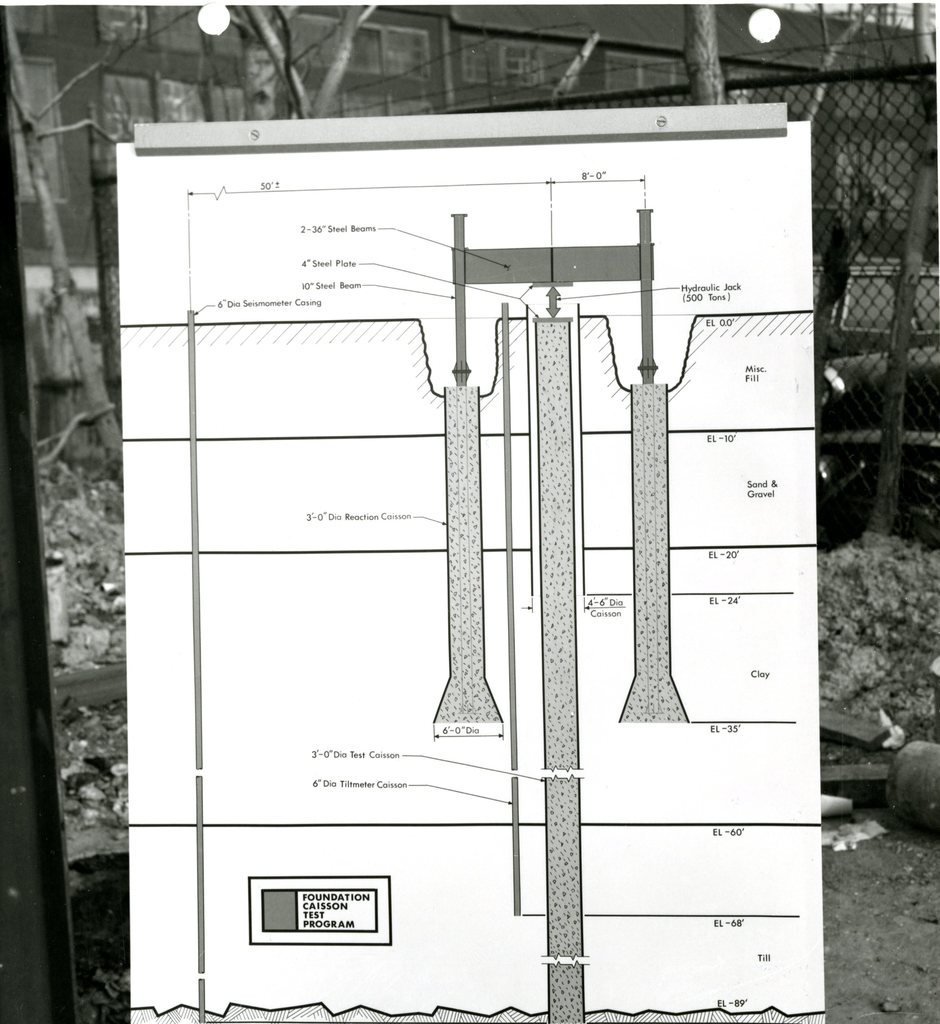
Specialized Foundations
Machine Foundations
Machine foundations must handle dynamic forces and vibrations. Concrete is commonly used in these foundations due to its high compressive strength and ability to dampen vibrations. Reinforced concrete also provides the necessary stiffness to resist oscillatory motions. The design often includes blocks, walls, or frames of concrete, depending on the machine type and operational conditions.
Bridge Foundations
Bridge foundations must withstand heavy loads and often challenging environmental conditions. Concrete is an ideal material with its high compressive strength and durability. Pile, pier, or caisson foundations are commonly used, all involving concrete to a large extent. For underwater foundations, the use of waterproof or marine concrete is common. Reinforcement in these foundations resists tensile forces and increases longevity.
Concrete Mixing, Placement, and Curing for Foundations
Concrete Mixing Principles for Foundation Work
The quality of concrete in foundation work is contingent upon the correct proportions of its constituents: cement, aggregates (coarse and fine), water, and any admixtures. This mix needs to be carefully tailored to provide the strength and workability needed for the foundation.
The water-cement ratio is particularly crucial. A lower ratio provides higher strength but decreases workability, making the concrete harder to pour and compact. Conversely, a higher ratio increases workability but reduces strength. Thus, an optimal balance is required, often achieved using admixtures to maintain workability without compromising strength.
The aggregates must be clean, hard, and appropriately graded. Fine aggregates or sand fills in the spaces between the coarse aggregates, and cement paste binds these aggregates together. Properly graded aggregate ensures less void space, requiring less cement paste, leading to a more economical and durable concrete mix.
Moreover, consistency in mixing is key to ensuring homogeneity and uniformity, leading to the best structural performance. All these considerations ensure a concrete mix suitable for foundation work, providing the desired strength, durability, and workability.
Placement Techniques for Concrete Foundations
Concrete placement in foundations must be done carefully to ensure a homogenous and void-free layer, providing the necessary strength and stability. Here are the key steps:
Preparation: The site should be prepared by excavating to the required depth, ensuring the soil is compact, and placing the formwork correctly. Any reinforcement bars should be correctly positioned.
Pouring: Concrete should be poured continuously to avoid cold joints, which can weaken the structure.
Compaction: The concrete should be compacted as soon as it is poured, using mechanical vibrators. This step is crucial to eliminate air bubbles and ensure the concrete is compacted into every corner of the formwork.
Levelling: The concrete should be levelled off at the top of the formwork using a straightedge.
Curing Process and Its Importance in Foundation Integrity
Curing is a critical step in concrete placement that significantly impacts the strength and durability of the finished product. It involves maintaining adequate moisture and temperature conditions over a period to allow the hydration reaction of cement to continue until the desired strength is achieved.
For foundations, proper curing is crucial. If the concrete dries too quickly, the hydration process will be incomplete, leading to lower strength and potentially resulting in cracks, reducing the foundation’s integrity.
There are several methods of curing, such as water curing (ponding or immersion), membrane curing (using a sealing compound), and steam curing (for precast concrete). The choice of method depends on the specific conditions and requirements.
Curing should typically start as soon as the concrete has hardened enough to resist surface damage and continue for a minimum of 7 days for ordinary Portland cement. This process ensures that the concrete achieves the desired properties, ensuring a durable and reliable foundation.
Modern Innovations in Concrete for Foundation Design
High-Performance Concrete
High-performance concrete (HPC) is an innovative material designed to have superior mechanical and durability properties compared to standard concrete. It often includes additives like silica fume or fly ash, and its characteristics can be tailored to meet specific needs. For foundations, HPC provides high compressive strength, ensuring greater load-bearing capacity. It also displays enhanced durability, reducing the effects of environmental degradation and ensuring the foundation’s longevity. Using HPC can lead to longer service life and lower maintenance costs, making it a preferred choice for critical infrastructure.
Self-Compacting Concrete
Self-compacting concrete (SCC) is another significant innovation. This type of concrete can flow and compact under its weight, eliminating the need for mechanical vibration. This feature is particularly useful in foundations with complex formwork or dense reinforcement, where traditional compaction might be challenging. SCC provides a homogenous and void-free concrete layer, leading to improved strength and durability. It also increases work efficiency and safety by reducing the labor involved in compaction.
Fibre-Reinforced Concrete
Fibre-reinforced concrete (FRC) incorporates small fibres distributed uniformly throughout the concrete mix. The fibres, which can be made of steel, glass, or synthetic materials, provide enhanced tensile and flexural strength, reducing the risk of cracking and improving the durability of the foundation. This property is particularly beneficial in areas prone to ground movement or seismic activity. FRC can also resist impact loads better than traditional concrete, making it suitable for foundations of structures subjected to such loads.
Applications and Case Studies
These innovations have seen successful applications across a variety of foundation projects. For instance, HPC has been used in the foundations of skyscrapers and bridges, providing the needed strength and durability. SCC has proven useful in constructing the foundations of power plants and other industrial structures with complex reinforcement patterns. FRC has been used in the foundations of buildings in earthquake-prone areas, improving the resilience of the structures. Detailed case studies, such as the use of HPC in the Burj Khalifa’s foundation or SCC in the Akashi Kaikyo Bridge, highlight the benefits of these innovative concretes in contemporary foundation design.
Ensuring the Longevity of Concrete Foundations
Causes of Foundation Failure
The causes of foundation failure can be broadly classified into design flaws, construction errors, and changes in the environmental conditions. Design errors may include inaccurate load calculations, inadequate soil investigation, or poor selection of the foundation type. Construction errors might include improper concrete mixing, inadequate curing, or flawed placement of reinforcement. Environmental conditions like soil erosion, fluctuating groundwater levels, or seismic activity can also induce failure. Chemical attack from aggressive soils or waters on the concrete, leading to deterioration, is another significant factor. Over time, these issues can cause a loss of strength, cracks, or excessive settlement, signaling foundation failure.
Preventive Measures and Maintenance
Preventing foundation failure begins with a thorough site investigation, proper design, and good construction practices. The site investigation should accurately determine the soil properties to ensure the foundation is designed to handle the site conditions. The design should accurately calculate loads and consider factors like possible groundwater fluctuation or seismic activity. During construction, correct mixing, placement, and curing of concrete should be ensured.
Regular maintenance and inspections are crucial in the early detection of issues. Drainage systems should be maintained to prevent soil erosion or water accumulation near the foundation. Any cracks or signs of distress in the structure should be promptly investigated. Implementing these preventive measures and diligent maintenance can significantly enhance the longevity of concrete foundations.
Repair and Rehabilitation Techniques
If a foundation shows signs of distress, repair and rehabilitation techniques can be employed to restore its capacity. The choice of method depends on the extent and cause of the damage. Small cracks might be sealed using epoxy injection, while larger cracks or settlements might require more extensive methods like underpinning, which involves adding additional support below the existing foundation. In severe cases, the foundation might need to be replaced entirely.
Surface treatments or coatings can provide a protective barrier in case of concrete deterioration due to chemical attack. In other cases, techniques like jacketing, where a new layer of reinforced concrete is added around the existing foundation, can be employed to increase strength and durability.
Combined with preventive measures, these techniques can ensure the longevity of concrete foundations, contributing to the safe and long-lasting performance of the overall structure.
Environmental and Sustainability Considerations
Environmental Impact of Concrete Production
Concrete production significantly contributes to global CO2 emissions, primarily due to the cement manufacturing process, which involves the calcination of limestone. Moreover, the extraction of raw materials like sand and aggregates impacts local ecosystems and can lead to resource depletion.
Water pollution is another concern, as the washing and disposal of concrete production waste can contaminate water bodies. The consumption of large quantities of water in concrete production also puts a strain on this vital resource.
Energy consumption during the production and transportation of concrete also contributes to its environmental footprint. Moreover, concrete structures have a long lifespan, and their demolition leads to significant waste generation.
Sustainable Practices in Concrete Foundation Construction
Sustainable practices in concrete foundation construction can mitigate some of these environmental impacts. These practices include using supplementary cementitious materials like fly ash or slag to replace part of the cement, reducing CO2 emissions. Recycled aggregates can be used to reduce the demand for natural resources.
Water recycling systems can be implemented in concrete plants to reduce water consumption and pollution. Energy-efficient machinery and local sourcing of materials can reduce energy consumption and transportation-related emissions.
Waste management practices, such as reusing or recycling concrete waste, can also contribute to sustainability. The design of foundations should also consider the lifespan and future demolition of the structure, promoting a circular economy approach.
Future Trends and Green Innovations in Concrete Foundations
Innovation is driving the development of greener concrete alternatives for foundations. Low-carbon concrete, which uses novel cements with a significantly lower carbon footprint, is one promising trend.
The use of natural and recycled fibers in fiber-reinforced concrete can reduce environmental impact and improve performance. Additionally, self-healing concrete, incorporating bacteria or microcapsules that release healing agents when a crack forms, promises longer-lasting and more sustainable structures.
Carbon capture and utilization in concrete production is another exciting field. Some companies are developing technologies to use captured CO2 to produce synthetic aggregates or even to cure concrete, storing the CO2 permanently.
These green innovations, combined with sustainable practices, hold the potential to significantly reduce the environmental impact of concrete foundations, contributing to more sustainable infrastructure development.
Case Studies
Notable Examples of Concrete Foundations in Architecture
Several noteworthy architectural projects highlight the versatility and strength of concrete foundations:
Burj Khalifa, Dubai: The foundation of the world’s tallest building consists of a reinforced concrete mat, which is 3.7 meters thick and supported by 192 bored piles. The use of high-performance concrete ensures the foundation’s ability to support the immense load.

Millau Viaduct, France: This bridge, the world’s tallest, relies on deep pile foundations going as deep as 15 meters into the ground. The use of concrete in these foundations provides the necessary strength and stability.
The Shard, London: The foundation of Western Europe’s tallest building is top-down, where the basement floors and foundation piles are constructed simultaneously. The use of concrete ensured the foundation’s durability and strength, despite the challenging conditions of the project. The foundation consists of 120 drilled piles that are four feet wide in diameter and 177 feet deep. A 13 foot mat foundation is included at a depth of 52 feet.
Lessons Learned and Best Practices
From these case studies, we can derive several best practices for designing and constructing concrete foundations:
Thorough Site Investigation: A comprehensive site investigation was conducted to determine the optimal foundation type and the required concrete properties. This practice ensures the foundation suits the soil conditions and supports the imposed loads.
Use of High-Performance Concrete: High-performance concrete was used in these projects to meet the unique strength and durability requirements. This practice underlines the importance of tailoring the concrete properties to the project’s specific needs.
Quality Control in Construction: Rigorous quality control was maintained during construction, ensuring correct concrete mixing, placement, and curing. This practice is crucial in achieving the desired properties and performance of the foundation.
Innovation and Flexibility: Each project used innovative and flexible approaches, adapting to the project’s unique challenges. This practice shows the importance of innovative thinking and flexibility in achieving successful outcomes in complex foundation projects.
Conclusion
The use of concrete in foundation design underpins much of modern architecture. With its adaptable properties and substantial strength, concrete has proven indispensable in supporting structures of all sizes and purposes. The role of concrete in the diverse range of foundation types, from shallow to deep foundations, underscores its universal application. Additionally, the process of concrete mixing, placement, and curing, specifically tailored for foundations, exemplifies the critical role of concrete in ensuring structural integrity. The innovations in concrete types, whether high-performance, self-compacting, or fiber-reinforced, demonstrate concrete’s ongoing evolution to meet architectural challenges and demands.
Looking ahead, the use of concrete in foundation design is set to embrace more sustainable practices and green innovations. The focus on reducing the environmental impact of concrete production, combined with innovations such as low-carbon concrete and self-healing concrete, will transform how foundations are designed and constructed. Moreover, as the architectural world grapples with the challenges of climate change and resource scarcity, the role of concrete in foundation design will continue to evolve. Ultimately, the future of concrete foundations lies in balancing the structural needs of architecture with the pressing environmental imperatives of our time, ensuring the sustained viability of our built environment.