6 History and Properties of Wood and Lumber
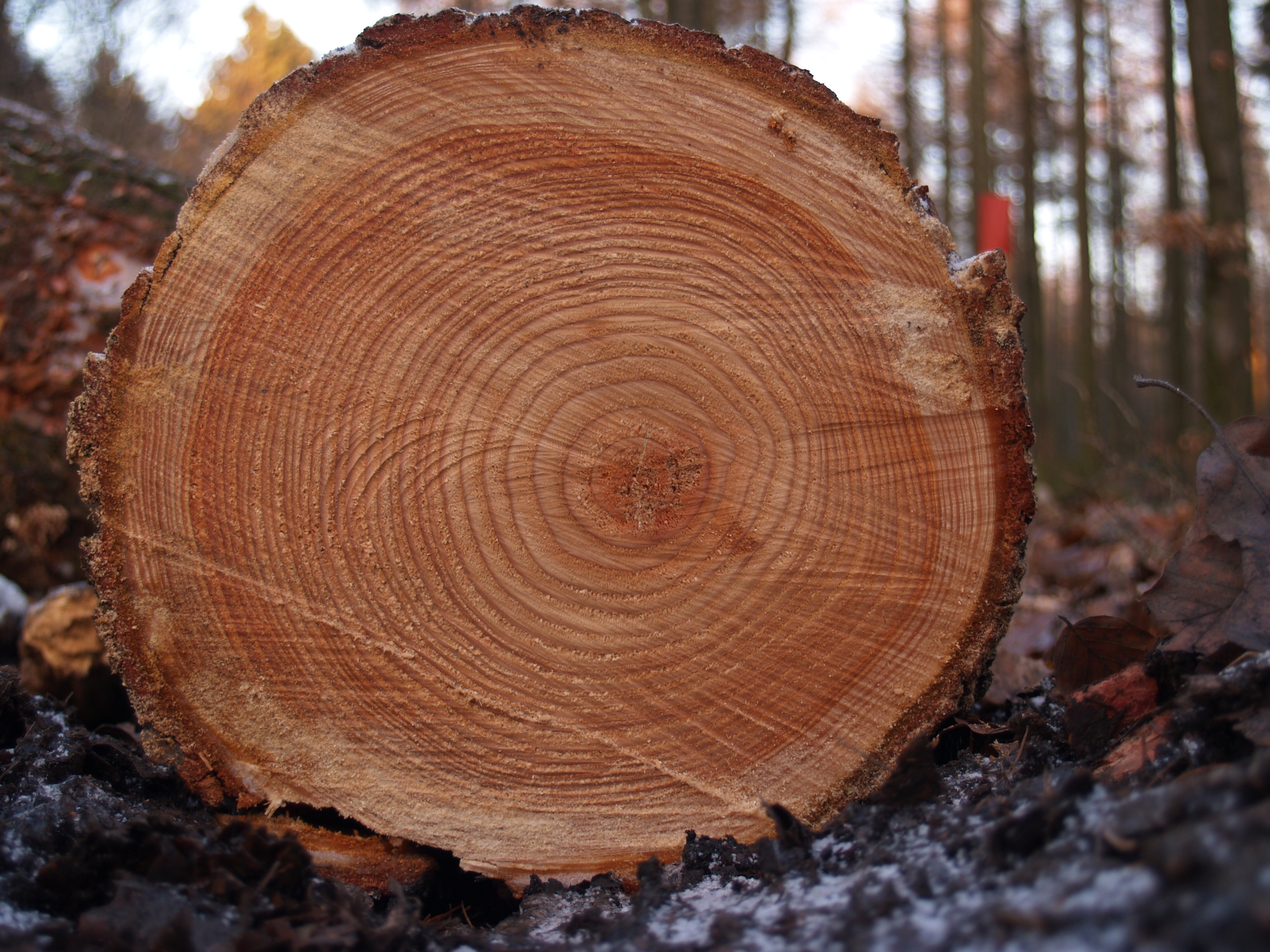
Introduction
History of Wood Use
Prehistoric Era
Wood, as a versatile and readily available resource, has played an essential role since the dawn of humanity. Our prehistoric ancestors recognized its utility and harnessed its potential in a myriad of ways.
Earliest known use of wood
The earliest known use of wood dates back to roughly 1.7 million years ago in the early Pleistocene era, as suggested by archaeological evidence. Early hominins, like Homo habilis, started using wood for various purposes, such as fuel and shelter. In particular, wood was a primary source of fuel for the fire, an elemental discovery that played a significant role in the evolution of early humans. Fire brought warmth and protection from predators and opened up a new way to consume food—cooked rather than raw. These benefits, in turn, enabled early humans to colonize colder climates and evolve complex social structures around the hearth.
Introduction of wood in tools and weapons
As Homo habilis evolved into Homo erectus, the use of wood became more sophisticated. Early hominins discovered that wood could be shaped into tools and weapons, setting the stage for technological advancements that would shape human destiny. The earliest wooden tools were likely simple branches and logs used for digging and foraging.
By the time of Homo erectus, around 1.6 million years ago, evidence suggests a leap in tool-making capabilities. Wooden spears from this period have been discovered at various archaeological sites. For example, the famous site of Schöningen in Germany has yielded wooden javelins that demonstrate a surprising degree of workmanship, hinting at the complex cognitive abilities of early humans.
This marked the beginning of an enduring relationship between humans and wood—a relationship characterized by constant innovation and discovery, propelling us from mere survival towards the early stages of civilization. These simple but crucial beginnings laid the groundwork for the extensive and varied uses of wood that we see in the following epochs, and continue to see today.
Ancient Civilizations
As we moved into the period of ancient civilizations, wood continued to be a cornerstone of human development. It was utilized in various forms, from construction material to a medium for artistic expression, underlining its importance in the growth of societies.
Egyptian use and import of wood
The Ancient Egyptians were well known for their ingenious use of wood, despite the scarcity of forests in Egypt. Due to limited local supplies, they imported wood from foreign lands, such as cedar from Lebanon and ebony and ivory from Africa. Cedar was prized for its durability and resistance to insects and was often used for construction, furniture, and shipbuilding. Artisans skillfully crafted wood into intricate furniture, statues, and even sarcophagi. The Ancient Egyptians also used wood in their revolutionary technique of papyrus-making, a precursor to modern paper.
Greek and Roman utilization of wood in construction
The Ancient Greeks and Romans, renowned for their architectural marvels, also employed wood extensively. Greek temples, originally made of wood, later influenced the stone columns that we associate with Greek architecture today. The Ancient Greeks revered oak because they believed it was sacred to Zeus, the king of the gods, and used it in construction and for making religious idols.
The Romans, with their advanced engineering and architectural skills, incorporated wood in a variety of structures such as houses, bridges, and aqueducts. Roman engineers made use of timber piles for foundational support in their infrastructure, including the famous Flavian Amphitheater, better known as the Colosseum. They also developed early forms of plywood, further underlining the inventive ways in which wood was used during this era.
Thus, wood played an instrumental role in these ancient societies, not only in their architectural accomplishments but also in their socio-cultural narratives, intertwining the material with myth, religion, and the growth of civilization.
Medieval to Modern Times
As humanity moved from antiquity into the medieval period and later into modernity, wood remained a quintessential material, adapting to each epoch’s changing needs and technologies.
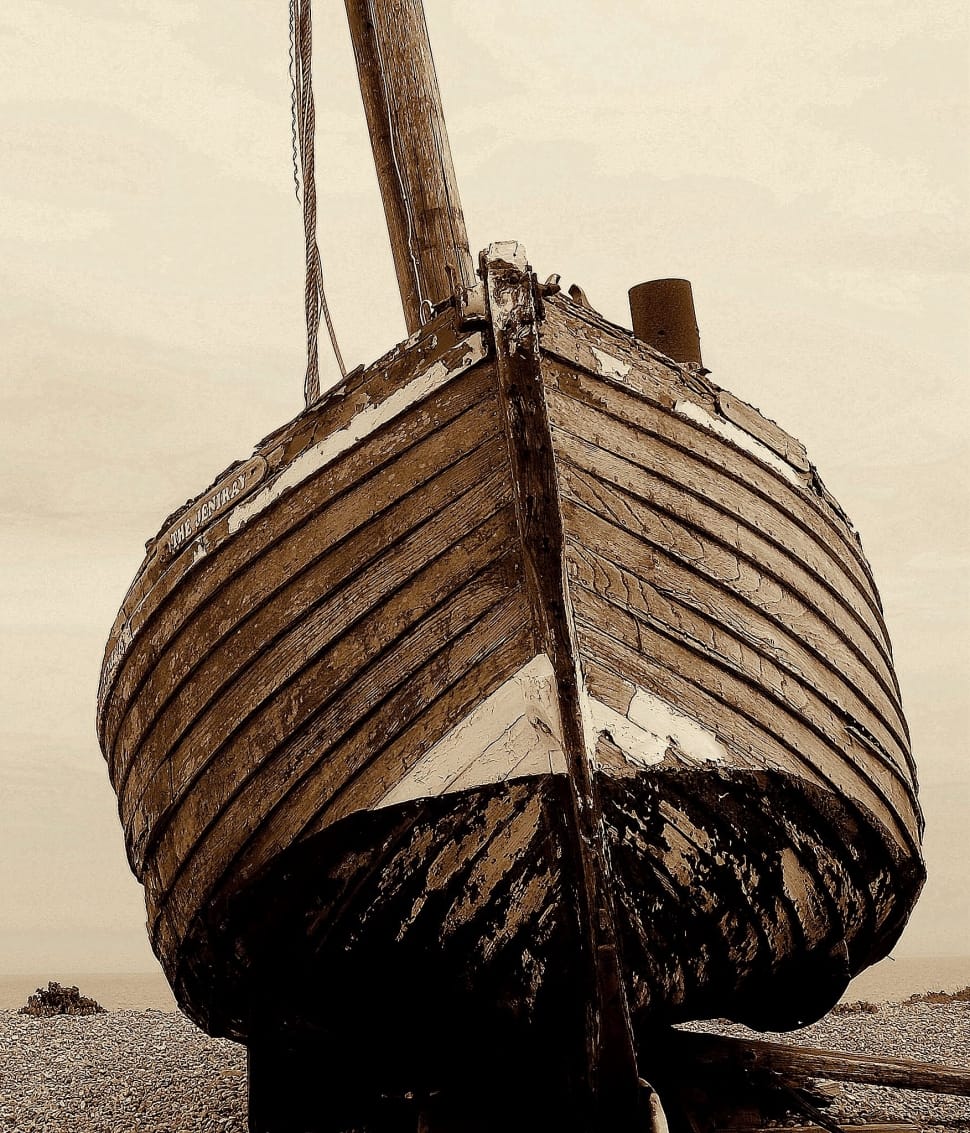
Wood in shipbuilding and architecture
During the Middle Ages, wood was prominent in shipbuilding and architecture. Wooden vessels, from the Viking longships to the caravels of the Age of Discovery, enabled trade, exploration, and conquest, fundamentally shaping the course of history. The Vikings, renowned for their seafaring abilities, built fast and durable longships from wood, facilitating their exploration and expansion across the North Atlantic.
In architecture, the medieval period witnessed the rise of timber framing, a technique that used heavy timbers jointed via intricate woodworking joints. Examples of this style, like the half-timbered houses in Germany and England or the pagodas in Japan, demonstrated the versatility and durability of wood as a construction material.
Industrial Revolution and the rise of the lumber industry
The advent of the Industrial Revolution in the late 18th century brought about significant changes in the use of wood. The development of steam power and, later, electrical machinery revolutionized the lumber industry. Sawmills became more efficient, enabling the production of lumber on a much larger scale. Wood was used extensively in the burgeoning railway industry for constructing rail cars and the railway ties that underpinned the vast network of tracks.
With the rise of mass production and the expansion of cities, the demand for wood increased dramatically, leading to a boom in the lumber industry. However, this also led to large-scale deforestation, introducing the need for sustainable forestry practices, a concern that continues to resonate in our contemporary world.
From the Middle Ages to the Industrial Revolution, wood’s role evolved significantly, mirroring these periods’ technological advancements and socio-economic changes. Its story is intertwined with the narrative of human progress, reinforcing its importance in our shared history.
Contemporary Wood Usage
As we moved into the 21st century, wood has retained its relevance in a world increasingly focused on sustainability and environmental responsibility.
Role of wood in modern construction
In contemporary construction, wood has proven to be an extraordinarily versatile material. Advances in wood processing and engineering have led to the development of innovative products like cross-laminated timber (CLT), glue-laminated timber (glulam), and laminated veneer lumber (LVL), to name a few. These engineered wood products combine wood’s natural beauty and biophilia with strength and durability that rival traditional construction materials like steel and concrete.
Wood is extensively used in residential construction for framing, flooring, and cabinetry. Moreover, the emergence of mass timber construction has reintroduced wood to large-scale, multi-story constructions. Often characterized by their beauty and sustainability, these structures exemplify the successful marriage of tradition and innovation.
Wood as a sustainable resource in the 21st century
In the era of climate change and environmental crises, the use of wood as a sustainable resource has taken on new significance. As a renewable material, wood stores carbon throughout its lifetime, helping to mitigate greenhouse gas emissions. When harvested responsibly from well-managed forests, wood provides a lower environmental impact alternative to more energy-intensive materials.
Additionally, innovative practices such as urban forestry and salvage logging have created new sources for wood materials, contributing to the circular economy and reducing waste.
The burgeoning field of biotechnology is also exploring the use of wood in surprising new ways, such as the development of transparent wood for windows or the use of nanocellulose, a derivative of wood pulp, in a variety of high-tech applications.
As we navigate the challenges and opportunities of the 21st century, wood continues to play a pivotal role in our lives. Its enduring relevance speaks volumes about its historical significance and its potential for the future, anchoring it as a material of immense importance in our shared journey.
Fundamental Properties of Wood
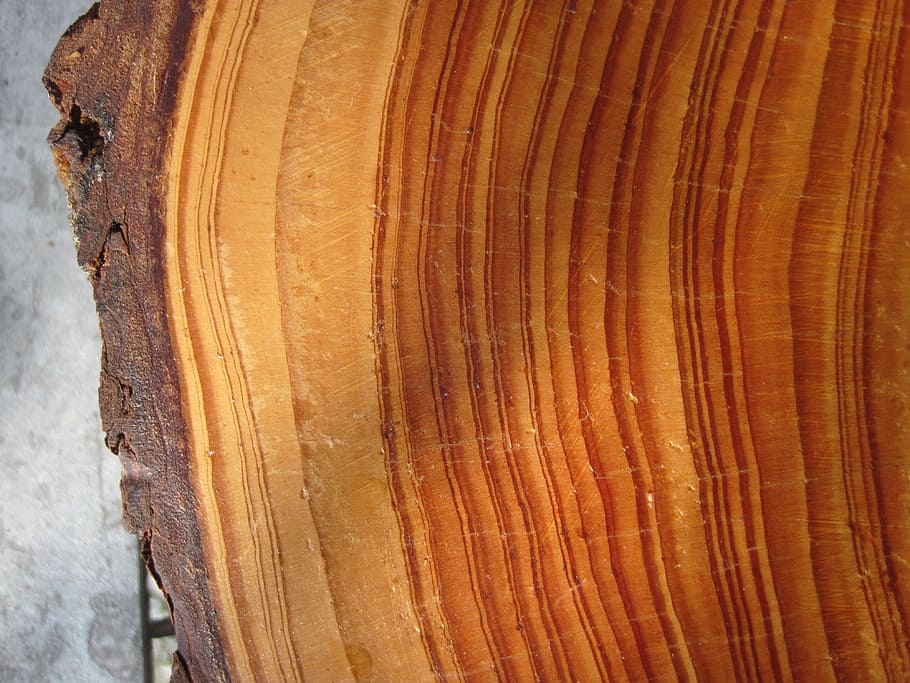
Anatomical Structure of Wood
Understanding the fundamental properties of wood requires a close look at its anatomical structure. This microscopic architecture gives wood its characteristic properties and shapes its interactions with the surrounding environment.
Cell structure and its impact on properties
Wood is essentially a mass of cells, primarily made up of three types: parenchyma, fibers, and vessels or tracheids. Each of these cells plays a specific role and contributes to the overall properties of wood.
Parenchyma cells, generally arranged in longitudinal and radial patterns, store and distribute nutrients. Fibers are elongated cells that contribute to the mechanical strength of wood, especially its compressive and tensile strength. Vessels in hardwoods, and tracheids in softwoods, are responsible for conducting water from the roots to the leaves.
The cell walls of these cells are composed of cellulose, hemicellulose, and lignin. Cellulose gives wood its tensile strength, hemicellulose binds cellulose fibers together, and lignin, the primary component responsible for the rigidity of wood, provides compressive strength and resistance to decay.
These cells’ arrangement, density, and cell wall composition significantly affect the wood’s physical and mechanical properties, such as hardness, strength, density, and shrinkage.
Variations in wood grain and growth rings
Wood grain refers to wood fibers’ alignment, texture, and appearance. Grain patterns arise from the orientation of the wood’s cells and growth rings, and they can greatly affect the working properties of wood, including how it responds to being cut, planed, or shaped.
Growth rings, visible in a cross-section of a tree trunk, represent the variations in growth rate between the seasons. Each ring comprises an outer lighter section, the earlywood, which grows in the spring and early summer, and an inner darker section, the latewood, which grows later in the summer and fall. The number of growth rings per inch, and the proportion of earlywood to latewood, impacts the wood’s density, weight, strength, and hardness.
By understanding the cellular structure of wood and the intricacies of grain patterns and growth rings, we can better predict and manipulate wood’s properties for a diverse range of applications. This knowledge also helps us appreciate the complexity and adaptability of this vital natural resource.
Physical Properties of Wood
The physical properties of wood arise from its cellular structure and determine its functionality in a variety of applications.
Density and hardness
The density of wood, typically measured as the mass per unit volume, significantly influences its mechanical properties, such as strength and hardness. The growth rate of the tree, cell wall thickness, and the proportion of latewood in growth rings primarily determines it. Denser woods, which contain a higher proportion of latewood, are generally harder and stronger but also heavier. Hardness is usually evaluated by resistance to indentation tests, and it is a crucial factor in applications such as flooring or tool handles where wear resistance is important.
Thermal properties, electrical properties, acoustic properties
Wood is an excellent insulator due to the presence of tiny air pockets within its cellular structure. This characteristic, coupled with its low thermal conductivity, makes wood an effective material for construction in both hot and cold climates.
The electrical properties of wood, like electrical resistivity, are influenced by factors such as moisture content, temperature, and grain direction. Generally, wood is a poor conductor of electricity, which is beneficial in certain applications like utility poles.
Wood’s acoustic properties, including sound absorption and sound velocity, make it a favorite choice for musical instruments like guitars and violins. The unique cellular structure of wood allows it to absorb sound and minimize echo, enhancing sound quality. Moreover, wood’s natural resonance contributes to the warm, rich tones produced by wooden musical instruments.
By understanding these physical properties, we can select the appropriate wood for a given application, whether that’s for constructing energy-efficient buildings, crafting durable furniture, or producing beautiful music. Furthermore, these properties underscore wood’s versatility and adaptability, characteristics that have underpinned its central role in human development throughout history.
Chemical Properties of Wood
At the chemical level, wood consists of a complex matrix of organic compounds, primarily cellulose, hemicellulose, and lignin, that contribute to its structural integrity and determine its durability.
Cellulose, hemicellulose, and lignin composition
Cellulose, a linear polysaccharide polymer, is the primary structural component of the cell wall in wood. It contributes to wood’s mechanical strength and rigidity. Cellulose fibers embedded in a lignin and hemicellulose matrix give wood its strength, flexibility, and resilience properties.
Hemicellulose is a branched polymer that wraps around cellulose, enhancing the structural integrity of the cell wall. It also increases the capacity of wood to hold water, thus affecting its dimensional stability.
Lignin acts as a natural adhesive, binding cellulose fibers together and providing rigidity. It is primarily responsible for the compressive strength of wood and plays a crucial role in its resistance to decay.
The composition and distribution of these compounds vary among different species, contributing to each wood type’s unique characteristics and properties.
Durability and resistance to decay
The durability of wood, defined by its resistance to biological degradation, is closely linked to its chemical properties. While all wood is susceptible to decay under certain conditions, the degree of susceptibility varies among species. The heartwood of many species contains extractives – compounds like tannins, oils, resins, and alkaloids – that provide natural resistance to decay and insect attack.
However, even durable wood species are susceptible to decay under prolonged exposure to moisture, fungi, and insects. Consequently, wood preservation techniques such as pressure treatment with preservatives are often employed to enhance durability.
Understanding the chemical composition of wood allows us to appreciate the complex interplay of organic compounds that give wood its unique properties. It also provides insight into wood’s vulnerability to decay and the techniques we can employ to protect and preserve this valuable resource.
Biological Factors Affecting Wood Properties
Several biological factors, including the species of tree and environmental conditions, can significantly influence the properties of wood.
Impact of tree species on wood properties
The species of a tree largely determines the properties of its wood. For example, hardwoods (from angiosperm trees) and softwoods (from gymnosperm trees) possess different cellular structures, resulting in varying physical and mechanical properties. Hardwoods typically have a more complex structure and are generally denser and harder than softwoods, which have a simpler structure and are typically lighter and easier to work with.
There is considerable variation even within the broad categories of hardwoods and softwoods. The strength, hardness, density, color, grain pattern, and even smell of wood can vary widely from species to species. For example, balsa is a lighter and less dense hardwood than most softwoods. Conversely, yew is a softwood that is harder than many hardwoods.
Effects of environmental factors
Environmental factors such as soil quality, climate, altitude, and exposure to sunlight and wind can significantly affect wood properties. Trees grown in cold climates or at high altitudes generally grow slower, resulting in denser wood with closely spaced growth rings. Conversely, trees grown in warmer climates or fertile soils tend to grow faster, resulting in less dense wood with wider growth rings.
Environmental stress, such as wind or snow load, can result in a reaction wood—wood with altered properties that help the tree resist these stresses. In hardwoods, tension wood tends to form on the upper side of branches and leaning trunks. In softwoods it’s called compression wood and form on the lower side.
Pests, diseases, and fungi can also affect wood properties. For example, spalting—a coloration caused by certain fungi—can add unique patterns and colors to wood, often sought after in woodturning and decorative woodworking.
Understanding these biological factors helps us predict and manipulate the properties of wood, allowing us to use this versatile material more effectively and sustainably in a variety of applications.
Mechanical Properties of Lumber
The mechanical properties of lumber, such as strength and stiffness, are crucial considerations in its usage, especially in construction and structural applications.
Strength and stiffness
Strength refers to the maximum stress that lumber can withstand without failure, while stiffness (also known as modulus of elasticity) measures its ability to resist deformation under load. These properties are determined by factors such as wood species, grain direction, moisture content, and the presence of defects like knots or checks.
Hardwoods are generally stronger and stiffer than softwoods, although there is considerable variation among species. For example, oak is known for its exceptional strength and is commonly used in applications that require high load-bearing capacity, while pine, a softwood, is less strong but still suitable for many construction applications due to its relative stiffness and availability.
Factors affecting mechanical properties
Several factors influence the strength and stiffness of lumber:
Species: Different wood species have inherently different strengths and stiffness due to their cellular structure and chemical composition.
Grain direction: Wood is anisotropic, meaning its properties vary based on direction. Lumber is strongest and stiffest along the grain (longitudinally), less so across the grain (radially and tangentially).
Moisture content: As wood absorbs or loses moisture, its dimensions change, which can impact its strength and stiffness. Generally, lumber strength decreases and brittleness increases as it dries.
Defects: Knots, checks, splits, and decay can significantly reduce the strength and stiffness of lumber. This is why such defects are considered in the grading of lumber.
Understanding these mechanical properties allows for more efficient and safe use of lumber in structural applications, ensuring the integrity and longevity of structures from houses to bridges. As such, they form a crucial part of any discussion about the properties and uses of lumber.
Dimensional Stability of Lumber
The dimensional stability of lumber, or its ability to maintain its original dimensions under varying environmental conditions, is a vital characteristic that affects its performance and usability.
Shrinkage and swelling due to moisture changes
Wood is a hygroscopic material that absorbs or releases moisture until it reaches equilibrium with its surrounding environment. This moisture movement causes wood to shrink or swell, which can lead to changes in the dimensions of a piece of lumber.
Shrinkage typically occurs as wood dries and loses moisture. Like many wood properties, it is anisotropic, meaning it varies based on direction. It is most significant tangentially (along the growth rings), less so radially (across the growth rings), and least longitudinally (along the grain).
Conversely, swelling occurs when wood absorbs moisture. If unchecked, these dimensional changes can lead to warping, cracking, or other forms of distortion, which can affect the performance and aesthetics of wood products.
Techniques to improve stability
There are several techniques to improve the dimensional stability of lumber:
- Seasoning: This is the process of drying lumber to remove excess moisture. Seasoning can be done naturally (air-drying) or in controlled environments (kiln-drying). Properly seasoned wood is less likely to shrink or swell significantly during use.
- Wood treatments: Various chemical treatments can reduce wood’s hygroscopicity, thus improving its dimensional stability. These include treatments with water-repellent preservatives or impregnation with bulking agents that fill cell lumina, limiting their ability to absorb water.
- Engineered wood products: These products, such as plywood, laminated veneer lumber (LVL), or cross-laminated timber (CLT), are designed to limit dimensional changes. By gluing layers of wood with alternating grain direction, the natural movements of wood are counterbalanced, resulting in more stable products.
By understanding and managing the dimensional stability of lumber, we can ensure that it performs well in its intended application, whether it’s forming the framework of a house, crafting fine furniture, or producing a musical instrument. The quest for stability is a continual challenge in the use of this naturally variable material, but it is one that, when met, allows for the effective and sustainable use of this incredible resource.
Processing and Treatment of Lumber
The journey of wood from tree to finished lumber involves several processing and treatment steps that significantly influence the final properties and usability of the lumber.
Sawing, drying, and preservative treatments
Sawing: Once a tree is harvested, it is sawn into logs and then into rough lumber. The sawing pattern can influence the lumber’s grain pattern, strength, and stability. Common sawing methods include plain sawing (also known as flat sawing or through and through), quarter sawing, and rift sawing.
Drying: After sawing, the lumber is dried to reduce its moisture content and improve its stability and strength. This can be done through air drying or kiln drying. Kiln drying is faster and gives more control over the final moisture content, which is usually targeted to match the conditions where the wood will be used.
Preservative treatments: Lumber can be treated with various preservatives to enhance durability and resistance against decay and insect attack. These treatments are especially important for lumber used in exterior applications or in contact with the ground. Methods include pressure treatment, where the preservative is forced into the wood, and non-pressure treatments like brushing, spraying, or dipping.
Impact of processing and treatments on properties
The processing and treatment of lumber can greatly affect its properties:
Sawing influences the appearance (grain pattern), warp resistance, and sometimes the strength of the lumber. For instance, quarter-sawn wood typically shows a straighter grain pattern and is more stable (less prone to cupping) but yields less lumber per log and therefore is more expensive.
Drying enhances the dimensional stability of wood and improves its strength and hardness. However, drying must be carefully controlled to prevent defects like warping, splitting, or checking.
Preservative treatments enhance the durability of lumber, making it suitable for use in harsher environments. However, some treatments can affect the color or odor of the wood, and precautions must be taken when handling and disposing of treated wood due to the chemicals used.
Understanding these processing and treatment steps allows us to appreciate the complexities involved in producing high-quality lumber that meets the diverse needs of consumers and industry. They represent a key aspect of the link between the natural properties of wood and the tailored characteristics of the lumber products we use every day.
Future Trends in Wood and Lumber Use
As we look to the future, sustainability and efficient resource use have become vital in all industries, including forestry and lumber production. Wood is a renewable resource, but only if managed responsibly.
Sustainable Forestry Practices
Sustainable forestry practices aim to ensure that the extraction of timber products minimizes damage to the rest of the ecosystem. This involves careful management of the forest’s growth and regeneration, biodiversity, and ecological resilience.
Importance of sustainable practices
Sustainable practices are important to maintain the health and diversity of our forests, which are essential to many forms of life and perform vital ecological functions such as carbon sequestration and oxygen production. Forests also provide habitats for wildlife, contribute to local economies, and offer recreation opportunities.
Further, maintaining a sustainable source of timber is crucial for industries that depend on wood and lumber. If forests are over-exploited, it can lead to deforestation, loss of biodiversity, and a decline in available timber resources. Sustainable practices aim to balance the need for wood with the preservation of forest ecosystems.
Advances in forestry management
Modern sustainable forestry practices involve a mix of strategies, such as selective logging, where only certain trees are removed, allowing the forest to regenerate naturally, and plantation forestry, where trees are farmed specifically for timber production.
Technological advances, including remote sensing and Geographic Information Systems (GIS), now allow for more accurate mapping and monitoring of forest resources, improving our ability to manage them sustainably.
There is also a growing recognition of the importance of maintaining forest biodiversity, not only for the health of the ecosystem but also for the resilience of the forest and the quality of the timber resource.
Certification schemes, such as the Forest Stewardship Council (FSC) and the Programme for the Endorsement of Forest Certification (PEFC), have been established to promote and verify sustainable forestry practices. These certifications allow consumers to choose products from sustainably managed forests, driving demand for responsible practices.
The challenge for the future will be to continue to improve these sustainable forestry practices and to balance the need for timber resources with the health of our global forest ecosystems. This is essential to ensuring that wood and lumber continue to be valuable, renewable resources for generations to come.
Innovative Wood and Lumber Products
Innovation in the wood and lumber industry opens up new applications for this ancient material and offers potential solutions to contemporary global challenges.
Engineered wood products
Engineered wood products are a significant development in the use of wood. These products maximize the strength and utility of wood by manipulating its natural properties through various processes.
For example, Glulam (glued laminated timber) combines layers of lumber, each layer glued to its neighbor, to create a single, large, strong component that can be used in building structures. Cross-Laminated Timber (CLT) is a similar product that layers wood at right angles, enhancing dimensional stability and enabling the construction of large, pre-fabricated sections of buildings.
Engineered wood products like these allow for the construction of taller and larger wooden buildings than ever before, offering a sustainable alternative to steel and concrete in many applications.
Biomaterials and bioenergy from wood
In addition to traditional and engineered lumber products, wood is being used to produce a wide range of biomaterials and bioenergy. For instance, cellulose from wood is used to create a variety of products, including textiles, insulation, and biodegradable plastics. Lignin, a byproduct of the pulp and paper industry, can be used to produce biofuels and a variety of other materials.
Wood is also a significant source of renewable energy. It can be directly burned for heat or processed into pellets for more efficient combustion. In addition, technologies such as gasification and pyrolysis convert wood into gaseous or liquid fuels.
These innovations are creating new markets for wood and contributing to a bio-based economy that can help reduce reliance on fossil fuels and mitigate climate change.
The future of wood and lumber use will undoubtedly continue to evolve as we develop new technologies and strive to meet sustainability goals. Wood has been a trusted material for thousands of years, and with careful management and innovative thinking, it will continue to serve us for many years to come.
Conclusion
Throughout this chapter, we’ve journeyed from the prehistoric era to the present day, tracing the role of wood and lumber in human civilization. We’ve learned that wood, in its myriad forms, has been an essential component of human progress. It’s helped us build shelter, create tools, explore the world, and advance technologically. Today, as we face global challenges like climate change and resource scarcity, wood – when responsibly sourced and used – stands out as a sustainable, versatile, and high-performance material.
We’ve delved into the fundamental properties of wood, exploring its anatomical structure, physical and chemical properties, and the biological factors that impact these properties. Understanding these aspects of wood is crucial for its utilization in various applications, from construction to furniture making and from paper production to energy generation.
In the context of lumber, we’ve looked at grading standards, mechanical properties, dimensional stability, and processing techniques. These are important to appreciate the complexities involved in producing high-quality lumber that meets the diverse needs of consumers and industries.
We’ve also glimpsed the future of wood and lumber use, where sustainable forestry practices, innovative wood products, and new applications in biomaterials and bioenergy offer exciting possibilities. These trends point towards a future where wood and lumber continue to play pivotal roles in a sustainable, bio-based economy.
As you continue your studies, we encourage you further to explore the fascinating world of wood and its properties. The more we understand about this remarkable material, the better we can use it to meet our needs and protect the ecosystems that produce it. In this way, we can ensure that wood continues to be a vital, renewable resource for future generations. The history of wood and lumber use is far from over; its most exciting chapters may yet be unwritten.
References:
1. Hoadley, R. B. (2000). Understanding Wood: A Craftsman’s Guide to Wood Technology. Taunton Press.
2. Fridley, K.J. (1996). Designing with Wood: Unique Properties Require Unique Considerations. Civil Engineering, 66(11), 46–49.
3. Bowyer, J., Shmulsky, R., Haygreen, J. (2007). Forest Products and Wood Science: An Introduction. Blackwell Publishing.
4. Farjon, A. (2005). A Monograph of Cupressaceae and Sciadopitys. Royal Botanic Gardens, Kew.
5. FAO. (2010). Global Forest Resources Assessment 2010. Food and Agriculture Organization of the United Nations.
6. Upton, B., Miner, R. (2005). The Greenhouse Gas and Energy Impacts of Using Wood Instead of Alternatives in Residential Construction in the United States. Biomass and Bioenergy, 28(2), 215–226.
7. APA – The Engineered Wood Association. (n.d.). Retrieved from: https://www.apawood.org/
8. Forest Stewardship Council. (n.d.). Retrieved from: https://www.fsc.org/
9. Programme for the Endorsement of Forest Certification. (n.d.). Retrieved from: https://www.pefc.org/